Regulation of glucose in the body is done autonomically and constantly throughout each minute of the day. Normal BG levels should be between 60 and 140 mg/dL in order to supply cells of the body with its required energy. Brain cells don’t require insulin to drive glucose into neurons; however, there must still be normal amounts available. Too little glucose, called hypoglycemia, starves cells, and too much glucose (hyperglycemia) creates a sticky, paralyzing effect on cells. Euglycemia, or blood sugar within the normal range, is naturally ideal for the body’s functions. A delicate balance between hormones of the pancreas, intestines, brain, and even adrenals is required to maintain normal BG levels.
Fuels of the Body
To appreciate the pathology of diabetes, it is important to understand how the body normally uses food for energy. Glucose, fats, and proteins are the foods that fuel the body. Knowing how the pancreatic, digestive, and intestinal hormones are involved in food metabolism can help you understand normal physiology and how problems develop with diabetes.
Throughout the body, cells use glucose as a source of immediate energy. To keep the body running smoothly, a continuous concentration of 60 to 100 mg/dL of glucose in blood plasma is needed. During exercise or stress the body needs a higher concentration because muscles require glucose for energy (Basu et al., 2009). Of the three fuels for the body, glucose is preferred because it produces both energy and water through the Krebs cycle and aerobic metabolism. The body can also use protein and fat; however, their breakdown creates ketoacids, making the body acidic, which is not its optimal state. Excess of ketoacids can produce metabolic acidosis.
Functioning body tissues continuously absorb glucose from the bloodstream. For people who do not have diabetes, a meal of carbohydrates replenishes the circulating blood glucose about 10 minutes after eating and continues until about 2 hours after eating. A first-phase release of insulin occurs about 5 minutes after a meal and a second phase begins at about 20 minutes. Because the duration of insulin’s effect is only about 2 hours, taking a 2-hour postprandial (after meal) BG shows how well insulin was released and used by the body. The food is broken down into small components including glucose and is then absorbed through the intestines into the bloodstream. Glucose (potential energy) that is not immediately used is stored by the body as glycogen in the muscles, liver, and fat.
Your body is designed to survive and so it stores energy efficiently, as fat. Most Americans have excess fat because they replenish the glucose stores by eating before any fat needs to be broken down.
When blood glucose levels fall after 2 hours, the liver replenishes the circulating blood glucose by releasing glycogen (stored glucose). Glycogen is a polysaccharide, made and stored primarily in the cells of the liver. Glycogen provides an energy reserve that can be quickly mobilized to meet a sudden need for glucose.
Hormones of the Pancreas
Regulation of blood glucose is largely done through the endocrine hormones of the pancreas, a beautiful balance of hormones achieved through a negative feedback loop. The main hormones of the pancreas that affect blood glucose include insulin, glucagon, somatostatin, and amylin.
Insulin (formed in pancreatic beta cells) lowers BG levels, whereas glucagon (from pancreatic alpha cells) elevates BG levels.
Somatostatin is formed in the delta cells of the pancreas and acts as the “pancreatic policeman,” balancing insulin and glucagon. It helps the pancreas alternate in turning on or turning off each opposing hormone.
Amylin is a hormone, made in a 1:100 ratio with insulin, that helps increase satiety, or satisfaction and state of fullness from a meal, to prevent overeating. It also helps slow the stomach contents from emptying too quickly, to avoid a quick spike in BG levels.
As a meal containing carbohydrates is eaten and digested, BG levels rise, and the pancreas turns on insulin production and turns off glucagon production. Glucose from the bloodstream enters liver cells, stimulating the action of several enzymes that convert the glucose to chains of glycogen—so long as both insulin and glucose remain plentiful. In this postprandial or “fed” state, the liver takes in more glucose from the blood than it releases. After a meal has been digested and BG levels begin to fall, insulin secretion drops and glycogen synthesis stops. When it is needed for energy, the liver breaks down glycogen and converts it to glucose for easy transport through the bloodstream to the cells of the body (Wikipedia, 2012a).
In a healthy liver, up to 10% of its total volume is used for glycogen stores. Skeletal muscle cells store about 1% of glycogen. The liver converts glycogen back to glucose when it is needed for energy and regulates the amount of glucose circulating between meals. Your liver is amazing in that it knows how much to store and keep, or break down and release, to maintain ideal plasma glucose levels. Imitation of this process is the goal of insulin therapy when glucose levels are managed externally. Basal–bolus dosing is used as clinicians attempt to replicate this normal cycle.
While a healthy body requires a minimum concentration of circulating glucose (60–100 mg/dl), high chronic concentrations cause health problems and are toxic:
- Acutely: Hyperglycemia of >300 mg/dl causes polyuria, resulting in dehydration. Profound hyperglycemia (>500 mg/dl) leads to confusion, cerebral edema, coma, and, eventually, death (Ferrante, 2007).
- Chronically: Hyperglycemia that averages more than 120 to 130 mg/dl gradually damages tissues throughout the body and makes a person more susceptible to infections. The glucose becomes syrupy in the bloodstream, intoxicating cells and competing with life-giving oxygen.
The concentration of glucose in the blood is determined by the balance between the rate of glucose entering and the rate of glucose leaving the circulation. These signals are delivered throughout the body by two pancreatic hormones, insulin and glucagon (Maitra, 2009). Optimal health requires that:
- When blood glucose concentrations are low, the liver is signaled to add glucose to the circulation.
- When blood glucose concentrations are high, the liver and the skeletal muscles are signaled to remove glucose from the circulation.
Test Your Knowledge
Glycogen is:
- A hormone produced in the pancreas.
- A polysaccharide that is stored in the liver.
- Produced in the striated muscles when exercising.
- An energy reserve that is slow to mobilize in an emergency.
Apply Your Knowledge
If you want to lose weight, what fuel would you decrease in your diet and what fuels would you increase?
Online Resource
Video [2:36]
https://www.youtube.com/watch?v=OlHez8gwMgw
Answer: B
The Role of Insulin
Insulin is a peptide hormone made in the beta cells of the pancreas that is central to regulating carbohydrate metabolism in the body (Wikipedia, 2016). After a meal, insulin is secreted into the bloodstream. When it reaches insulin-sensitive cells—liver cells, fat cells, and striated muscle—insulin stimulates them to take up and metabolize glucose. Insulin synthesis and release from beta cells is stimulated by rising concentrations of blood glucose. Insulin has a range of effects that can be categorized as anabolic, or growth-promoting.
Functions of Insulin | |
---|---|
Turns on | Turns off |
Uptake and use of glucose by insulin-sensitive cells | Breakdown of glycogen in liver cells |
Storage of glucose in the form of glycogen in the liver and skeletal muscle tissue. Storage of fat. | Breakdown of fat |
Uptake of amino acids and the synthesis of proteins | Breakdown of protein |
DNA synthesis | Gluconeogenesis |
Test Your Knowledge
Insulin:
- Is only available by injection or orally to treat T2DM.
- Is a hormone that acts on the liver to convert excess glucose into glycogen.
- Inhibits the uptake and use of glucose by skeletal muscles.
- Is manufactured and secreted by the alpha cells of the pancreas.
Apply Your Knowledge
How would you explain the function of insulin to your patient with diabetes? What does it turn on and what does it turn off?
Answer: B
The Role of Glucagon
Glucagon, a peptide hormone secreted by the pancreas, raises blood glucose levels. Its effect is opposite to insulin, which lowers blood glucose levels. When it reaches the liver, glucagon stimulates glycolysis, the breakdown of glycogen, and the export of glucose into the circulation. In these ways, the effects of glucagon are catabolic, breaking down cells—the opposite of insulin’s anabolic effects (Drucker, 2008).
The pancreas releases glucagon when glucose levels fall too low. Glucagon causes the liver to convert stored glycogen into glucose, which is released into the bloodstream. High BG levels stimulate the release of insulin. Insulin allows glucose to be taken up and used by insulin-dependent tissues, such as muscle cells. Glucagon and insulin work together automatically as a negative feedback system to keeps BG levels stable.
Glucagon is a powerful regulator of BG levels, and glucagon injections can be used to correct severe hypoglycemia. Glucose taken orally or parenterally can elevate plasma glucose levels within minutes, but exogenous glucagon injections are not glucose; a glucagon injection takes approximately 10 to 20 minutes to be absorbed by muscle cells into the bloodstream and circulated to the liver, there to trigger the breakdown of stored glycogen.
People with type 2 diabetes have excess glucagon secretion, which is a contributor to the chronic hyperglycemia of type 2 diabetes. The amazing balance of these two opposing hormones of glucagon and insulin is maintained by another pancreatic hormone called somatostatin, created in the delta cells. It truly is the great pancreatic policeman as it works to keep them balanced.
Complementary Roles of Insulin and Glucagon
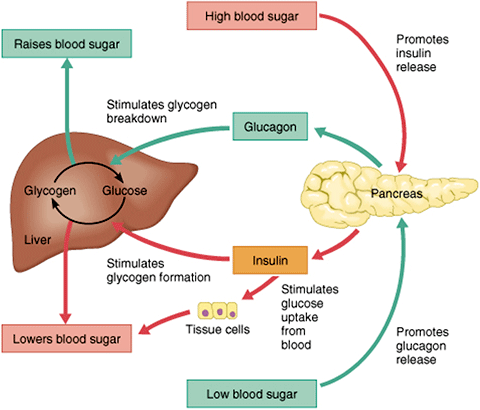
After you’ve eaten, the concentration of glucose in your blood rises. When it goes too high the pancreas releases insulin into the bloodstream. This insulin stimulates the liver to convert the blood glucose into glycogen for storage. If the blood sugar goes too low, the pancreas release glucagon, which causes the liver to turn stored glycogen back into glucose and release it into the blood. Source: Google Images.
Test Your Knowledge
Glucagon:
- Is a peptide hormone that is stored in the pancreas.
- Is used to treat hyperglycemia by increasing the uptake of glucose in muscles.
- Is a hormone that acts on the liver to convert glycogen back into glucose.
- Stimulates the production of insulin.
Apply Your Knowledge
How is glucagon available by injection?
Answer: C
The Role of Amylin
Amylin is a peptide hormone that is secreted with insulin from the beta cells of the pancreas in a 1:100 ratio. Amylin inhibits glucagon secretion and therefore helps lower BG levels. It also delays gastric emptying after a meal to decrease a sudden spike in plasma BG levels; further, it increases brain satiety (satisfaction) to help someone feel full after a meal. This is a powerful hormone in what has been called the brain–meal connection.
People with type 1 diabetes have neither insulin nor amylin production. People with type 2 diabetes seem to make adequate amounts of amylin but often have problems with the intestinal incretin hormones that also regulate BG and satiety, causing them to feel hungry constantly. Amylin analogues have been created and are available through various pharmaceutical companies as a solution for disorders of this hormone.
The Role of Incretins
Incretins are glucagon-like peptides (hormones) made in cells of the small intestine and secreted into the circulation in response to food intake (Cernea & Raz, 2011). Incretins go to work even before blood glucose levels rise following a meal. They also slow the rate of absorption of nutrients into the bloodstream by reducing gastric emptying, and they may also help decrease food intake by increasing satiety.
People with type 2 diabetes have lower than normal levels of incretins, which may partly explain why many people with diabetes state they constantly feel hungry. After research showed that BG levels are influenced by intestinal hormones in addition to insulin and glucagon, incretin mimetics became a new class of medications to help balance BG levels in people who have diabetes.
Two types of incretin hormones are GLP-1 (glucagon-like peptide) and GIP (gastric inhibitory polypeptide). Each peptide is broken down by naturally occurring enzymes called DDP-4, (dipeptidyl peptidase-4).
Exenatide (Byetta), an injectable anti-diabetes drug, is categorized as a glucagon-like peptide (GLP-1) and directly mimics the glucose-lowering effects of natural incretins upon oral ingestion of carbohydrates. The administration of exenatide helps to reduce BG levels by mimicking the incretins. Both long- and short-acting forms of GLP-1 agents are currently being used.
The functions of incretins are as follows:
- Stimulate insulin secretion
- Suppress glucagon secretion
- Slow gastric emptying to prevent spike in BG levels
- Increase satiety after a meal to signal to the brain to stop eating
Incretins are deactivated quickly by enzymes called DPP-4, in the bloodstream and on the surface of endothelial cells; thus, the glucose-lowering effects of incretins last only a few minutes (Drucker & Nauck, 2006). A new class of medications, called DPP4 inhibitors, block this enzyme from breaking down incretins, thereby prolonging the positive incretin effects of glucose suppression. An additional class of medications called dipeptidyl peptidase-4 (DPP-4 inhibitors—note hyphen), are available in the form of several orally administered products. These agents will be discussed more fully later.
Incretins Stimulate Insulin Release
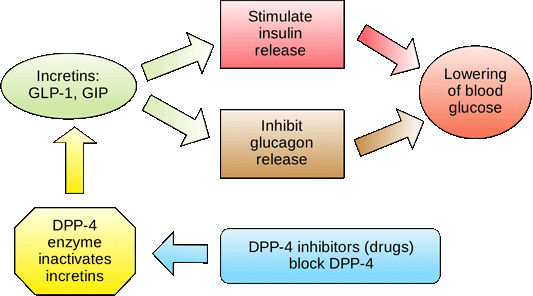
Source: Wikimedia Commons.
Poor Regulation of Blood Glucose
People with diabetes have frequent and persistent hyperglycemia, which is the hallmark sign of diabetes. For people with type 1 diabetes, who make no insulin, glucose remains in the blood plasma without the needed BG-lowering effect of insulin. Another contributor to this chronic hyperglycemia is the liver. When a person with diabetes is fasting, the liver secretes too much glucose, and it continues to secrete glucose even after the blood level reaches a normal range (Basu et al., 2009).
Another contributor to chronic hyperglycemia in diabetes is skeletal muscle. After a meal, the muscles in a person with diabetes take up too little glucose, leaving blood glucose levels elevated for extended periods (Basu et al., 2009).
The metabolic malfunctioning of the liver and skeletal muscles in type 2 diabetes results from a combination of insulin resistance, beta cell dysfunction, excess glucagon, and decreased incretins. These problems develop progressively.
Early in the disease the existing insulin resistance can be counteracted by excess insulin secretion from the beta cells of the pancreas, which try to address the hyperglycemia. The hyperglycemia caused by insulin resistance is met by hyperinsulinemia. Eventually, however, the beta cells begin to fail. Hyperglycemia can no longer be matched by excess insulin secretion, and the person develops clinical diabetes (Maitra, 2009).
Test Your Knowledge
People with type 2 diabetes have:
- Insulin sensitivity, which is an over-reaction of cells to insulin.
- No beta cells in their pancreas and no circulating insulin at all.
- Chronic hypoglycemia.
- Insulin resistance, which is a decreased response of cells to insulin.
Apply Your Knowledge
How would you explain to your patient what lifestyle behaviors create insulin resistance?
Answer: D
The Problem of Insulin Resistance
In type 2 diabetes, many patients have body cells with a decreased response to insulin known as insulin resistance. This means that, for the same amount of circulating insulin, the skeletal muscles, liver, and adipose tissue take up and metabolize less glucose than normal. Being less sensitive to insulin, the liver does not react to the usual signal of insulin, so the liver manufactures and secretes more glucose than is needed (Huether & McCance, 2012).
Insulin resistance can develop in a person over many years before the appearance of type 2 diabetes. People inherit a propensity for developing insulin resistance, and other health problems can worsen the condition. For example, when skeletal muscle cells are bathed in excess free fatty acids, the cells preferentially use the fat for metabolism while taking up and using less glucose than normal, even when there is plenty of insulin available. In this way, high levels of blood lipids decrease the effectiveness of insulin; thus, high cholesterol and body fat, overweight and obesity increase insulin resistance.
Physical inactivity has a similar effect. Sedentary overweight and obese people accumulate triglycerides in their muscle cells. This causes the cells to use fat rather than glucose to produce muscular energy. Physical inactivity and obesity increase insulin resistance (Monnier et al., 2009).
The Problem of Beta Cell Dysfunction
For people with type 1 diabetes, no insulin is produced due to beta cells destruction. Research shows this is an autoimmune response gone awry, attacking the body’s own cells. Triggers of that autoimmune response have been linked to milk, vaccines, environmental triggers, viruses, and bacteria.
For people with type 2 diabetes, a progressive decrease in the concentration of insulin in the blood develops. The continuously decreasing availability of insulin in type 2 diabetes is the direct result of a progressive worsening of the beta cells’ ability to produce enough insulin when it is needed (Huether & McCance, 2012).
Not only do the beta cells release less insulin as type 2 diabetes progresses, they also release it slowly and in a different pattern than that of healthy people (Monnier et al., 2009). Without sufficient insulin, the glucose-absorbing tissues—mainly skeletal muscle, liver, and adipose tissue—do not efficiently clear excess glucose from the bloodstream, and the person suffers the damaging effects of toxic chronic hyperglycemia.
At first, the beta cells manage to manufacture and release sufficient insulin to compensate for the higher demands caused by insulin resistance. Eventually, however, the defective beta cells decrease their insulin production and can no longer meet the increased demand. At this point, the person has persistent hyperglycemia. In type 2 diabetes, beta cells seemingly exhaust their capacity to adapt to the long-term demands of peripheral insulin resistance (Huether & McCance, 2012).
A downward spiral follows. The hyperglycemia and hyperinsulinemia caused by the over-stressed beta cells create their own failure. In type 2 diabetes, the continual loss of functioning beta cells shows up as a progressive hyperglycemia.
Test Your Knowledge
In type 2 diabetes:
- Beta cells in the pancreas cannot compensate for insulin resistance.
- The pancreas is attacked by the body’s immune system, resulting in pancreatitis.
- The liver becomes overly sensitive to insulin.
- Glucose cannot be used as fuel by any cells in the body.
Apply Your Knowledge
How would you explain insulin resistance differently to someone with type 1 diabetes and someone with type 2 diabetes?
Online Resource
Video [11:46]
https://www.youtube.com/watch?v=iTjDi2ZO0n8
Answer: A
Cell Damage in DM
Together, insulin resistance and decreased insulin secretion lead to hyperglycemia, which causes most of the health problems in diabetes. The acute health problems—diabetic ketoacidosis and hyperosmolar hyperglycemic state—are metabolic disorders that are directly caused by an overload of glucose. In comparison, the chronic health problems—eye, heart, kidney, nerve, and wound problems—are tissue injury, a slow and progressive cellular damage caused by feeding tissues too much glucose (ADA, 2015).
Hyperglycemic damage to tissues is the result of glucose toxicity. There are at least three distinct routes by which excess glucose injures tissues:
- Over time, excess glucose attaches to proteins in a process called glycosylation. For example, glycosylated hemoglobin (HbA1c), is the laboratory measure to monitor average glycemic levels. Glycosylated proteins trigger inflammatory reactions, which injure the lining of blood vessels. In addition, glycosylated proteins stick together on the basement membranes of capillaries, thickening the endothelial layers and disrupting their normal function.
- Excess intracellular glucose activates an enzyme called protein kinase C, which encourages the growth of unnecessary blood vessels, leads to blood vessel constriction, thickens basement membranes, and releases pro-inflammatory molecules such as C-reactive protein and homocysteine.
- Excess intracellular glucose reduces the effectiveness of the intracellular activities that protect against oxidants and oxidative stress. This leads to oxidative damage, especially in neurons. (Maitra, 2009)