Because an acquired neurologic injury (such as a stroke) affects both sensory and motor function, walking can be severely affected. Sensory changes, weakness, and spasticity affect movement strategies, which alter a person’s ability to successfully respond to losses of balance. A stroke affects how much and how often a person walks and also affects walking adaptability—the ability to adapt to different conditions during ambulation—as well as endurance. Gait training generally starts as soon as possible following a stroke, using manual techniques, task-specific training, strengthening, and, when available, body weight-assisted treadmill training and robotic devices.
Movement Strategies Altered by Stroke
A movement strategy or synergy is a flexible, repeatable pattern of movement that can be quickly and automatically accessed by the central nervous system. Movement strategies allow us to store and reuse patterns of movement that have been successful in the past. Strategies are efficient, automatic movement patterns that evolve over time. Each time a loss of balance threatens, the nervous system draws on these pre-programmed movement strategies to ensure the maintenance of balance.Movement strategies used by the nervous system to respond to perturbations are diminished following a stroke.
Ankle Sway
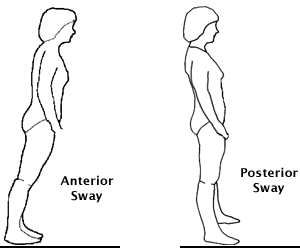
The ankle strategy is used in response to small perturbations is also called ankle sway. Source: Lauren Robertson.
The ankle strategy—also called ankle sway—is used in response to small perturbations or losses of balance. When a small loss of balance occurs—as when standing on a moving bus—the foot acts as a lever to maintain balance by making continuous automatic adjustments to the movement of the bus. When a small balance adjustment is needed, muscles close to the floor (anterior tibialis and gastrocnemius) activate first and flow upward in a distal to proximal pattern.
When a perturbation is too large to be successfully handled by the ankle strategy, the hip strategy is needed. When the hip strategy is used, movement is centered about the hip and ankle muscles (anterior tibialis and gastrocnemius) are almost silent. The muscles in the trunk activate first as activation flows downward to the legs in a proximal to distal pattern. So, if the bus stops suddenly and the body bends forward, the low back and hamstrings will contract in that order to return the body to upright.
If the perturbation is strong and your center of gravity moves well past your base of support, it is necessary to take a forward or backward step to regain balance. This is referred to as a stepping strategy. Studies have shown age-related changes in stepping in older adults. Compared to younger people, older adults initiate the stepping strategy in response to smaller losses of balance and tend to take several small steps rather than one larger step (Maki & McIlroy, 2006).
Arm movements have a considerable role in balance control and are part of the strategies discussed above. The upper limbs start to react at the very beginning of a disruption of balance and continue to be active as the body attempts to regain control. By automatically reaching and grasping for support, the arms perform a protective function. In the case of a small perturbation, upper limb movements can prevent a fall by shifting the center of gravity away from the imbalance.
When upper extremity paresis or spasticity is present, post stroke subjects exhibit poor protective reactions during a perturbation of balance. They demonstrate a deficit in anticipatory and reactive postural adjustments. These impairments of the affected upper limbs limit a person’s ability to recover from perturbations during functional tasks such as walking (Arya et al., 2014).
Even in the absence of a neurologic disorder, age-related changes affect upper extremity reaction time when balance is disrupted. Older adults reach for support surfaces more readily than younger adults but the reach-reaction time is slower. Increased tendency to reach for support and a slowing of these reactions have been found to be predictive of falling in daily activities (Maki & McIlroy, 2006).
Comparing Reflexes, Automatic Reactions, and Volitional Movement
Reflexes
Think for a moment that you are cooking dinner and accidentally touch a scalding hot fry pan. You feel the heat and withdraw instantaneously. You aren’t thinking “I better take my hand off the hot pan before it burns me”—your reflexes take care of that for you. The withdrawal is almost instantaneous because your nervous system senses danger and reflexively withdraws.
Automatic Reactions
This type of reaction is used in movement strategies; they are slower than reflexes but faster than volitional movement. They are fast enough to help us respond to losses of balance without having to think.
Volitional Movement
This type of movement requires thought and is relatively slow compared to reflexive and automatic movement. Using our brains to think about movement isn’t very practical when we need something done really fast—by the time your brain warns you to bend your waist, step forward, or grab onto something when the bus stops abruptly, it’s already too late to regain balance.
Activity
Stand up next to your chair. Make sure you are standing on a flat, firm surface. Now close your eyes. Notice that your body sways a little—you are using the ankle strategy to stay balanced. Notice also that after a short amount of time you sway less—that means your nervous system is adjusting. Often, following a stroke, a person looses the ability to use the ankle strategy. This can have a profound impact on balance.
Stand up again. Ask someone to give you a little nudge from behind. Try not to take a step. If it was a truly small nudge you will likely bend at the waist to try to regain your balance. This is an example of the hip strategy.
Now ask your partner to give you a slightly bigger nudge from behind. If the nudge is big enough you’ll have to take a step. This is the stepping strategy.
We use these strategies automatically, all day long, without effort. Someone who has had a stroke can’t access these strategies as quickly as you can. If faced with a nudge from a passerby, or a bus
Importance of Walking Early and Often
Regaining the ability to walk following a stroke is of paramount importance to patients and caregivers alike; improving balance and walking leads to greater independence and improves general well-being.
In the first week following a stroke, only one-third of patients are able to walk without assistance. In the following weeks, walking ability generally improves. At 3 weeks, or at hospital discharge, more than half of stroke survivors can walk unaided. By 6 months, more than 80% are able to walk independently without physical assistance from another person (Balasubramanian et al., 2014).
Following a stroke, walking can take a lot of energy; impaired muscle function, weakness, and poor cardiovascular conditioning can double the amount of energy expended. The high energy cost of walking can affect a person’s ability to participate in daily activities and lead to a vicious cycle where physical activity is avoided. For example, in one study, stroke patients walked 50% of the daily amount of matched sedentary adults and used 75% of their VO2 peak for walking at a submaximal rate (Danielsson et al., 2011).
Walking may improve more rapidly when patients are involved in setting specific goals. The results of several motor learning studies in which the person’s attention was focused on the outcome of an action rather than the action itself produced more effective performance than focusing on the quality of the movement (Carr & Shepherd, 2011).
In the hospital, an early goal for walking might be to walk to the next appointment, or to walk at least part of the way, rather than being transported in a wheelchair. Each day the patient should be encouraged to select a distance to walk independently and safely. Initially, this may be only a few steps. The goal is to walk the chosen distance a certain number of times a day, increasing distance as soon as possible, and keeping a record of progress, which gives the patient a specific focus (Carr & Shepherd, 2011).
Walking Adaptability, Stepping, and Postural Control
Walking is greatly dependent upon our ability to adapt to varying environmental conditions and tasks. Walking from the bedroom to the bathroom with a walker requires a different level of attention and adaptability than walking across a busy street carrying a bag of groceries. Even walking and talking can be a challenge for post stroke patients.
Over time, up to 85% of individuals with a stroke regain independent walking ability, but at discharge from inpatient rehab only about 7% can manage steps and inclines or walk the speeds and distances required to walk competently in the community. Limited ability to adjust to changes in the task and environment means a person either avoids walking in complex situations (a safety strategy) or has a heightened risk of falls when required to walk under these challenging conditions (Balasubramanian et al., 2014).
Despite its importance, assessment of walking adaptability has received relatively little attention. Frequently used assessments of walking ability after stroke involve walking short distances (such as the Timed Up and Go test) and examination of isolated limb movements (such as the Fugl-Meyer Assessment). Although valuable, these assessments do not take into account the skills needed to re-engage in safe and independent ambulation in the home and community. Comprehensive assessments and specific interventions are needed to improve walking adaptability (Balasubramanian et al., 2014).
In addition to the ability to adapt to different conditions and tasks, walking adaptability has two other requirements: (1) stepping, and (2) postural control (Shumway-Cook & Woollocott, 2012). Stepping involves the ability to generate and maintain a rhythmic, alternating gait pattern as well as the ability to start and stop. Postural control involves both the musculoskeletal and nervous systems.
To walk effectively, the central nervous system must:
- Generate the basic stepping pattern of rhythmic reciprocal limb movements while supporting the body against gravity and propelling it forward.
- Maintain control of posture (equilibrium) to keep the center of mass over a constantly moving base of support and maintain the body upright in space.
- Adapt to environmental circumstance or changes in the behavioral goal (Balasubramanian et al., 2014).
These components are especially necessary for complex tasks. For example, walking adaptability is crucial on uneven ground or cluttered terrains and when the task requires walking and turning or negotiating a curved path. There are endless combinations of task goals and environmental circumstances that must be considered to comprehensively capture walking adaptability (Balasubramanian et al., 2014).
Walking adaptability is very important for community ambulation. Patla and Shumway-Cook have described “dimensions” that affect a person’s ability to adapt while walking. These are external demands that must be met for successful community mobility:
- Distance (distance walked)
- Temporal factors (time needed to cross a busy street or crosswalk, ability to maintain the same speed as those around them)
- Ambient conditions (rain, heat, snow, etc.)
- Physical load (packages carried, number of doors that need to be opened)
- Terrain (stairs, curbs, slopes, uneven ground, grass, elevators, obstacles)
- Attentional demands (distractions in the environment, noise, cars, crowds, talking)
- Postural transitions (stopping, reaching, backing up, turning head, change direction)
- Traffic density (number of people within arm’s reach, unexpected collisions and near collisions with other people) (Shumway-Cook et al., 2002)
Improving Endurance for Walking
It is evident that many patients are discharged from inpatient rehabilitation severely deconditioned, meaning that their energy levels are too low for active participation in daily life. Physicians, therapists, and nursing staff responsible for rehabilitation practice should address this issue not only during inpatient rehabilitation but also after discharge by promoting and supporting community-based exercise opportunities. During inpatient rehabilitation, group sessions should be frequent and need to include specific aerobic training. Physical therapy must take advantage of the training aids available, including exercise equipment such as treadmills, and of new developments in computerized feedback systems, robotics, and electromechanical trainers.
Janet Carr and Roberta Sheperd
University of Sydney, Australia
Although many people affected by stroke have regained some ability to walk by the time they are discharged from rehab, many have low endurance, which limits their ability to perform household tasks or even to walk short distances. After a stroke, walking requires a much higher level of energy expenditure, and upon discharge many stroke patients are not necessarily functional walkers (Carr & Sheperd, 2011).
Functional walking is assessed using tests of speed, distance, and time. Minimal criteria for successful community walking include an independent walking velocity of 0.8 m/s or greater (about 2.6 feet/second), the ability to negotiate uneven terrain and curbs, and the physical endurance to walk 500 meters or more. In a review of 109 people discharged from physical therapy, only 7% achieved the minimum level. Cardiorespiratory fitness training can address both the efficiency with which people affected by stroke can walk and the distance they are able to achieve (Carr & Sheperd, 2011).
The loss of independent ambulation outdoors has been identified as one of the most debilitating consequences of stroke. Among stroke survivors 1 year after stroke, the most striking area of difficulty was low endurance measured by the distance walked in a 6-minute walk test. Those subjects able to complete this test were able to walk on average only 250 meters (820 feet) compared to the age-predicted distance of >600 meters (almost 2,000 feet), equivalent to 40% of their predicted ability and not far enough for a reasonable and active lifestyle. The detrimental effect of low exercise capacity and muscle endurance on functional mobility and on resistance to fatigue is likely to increase after discharge if follow-up physical activity and exercise programs are not available (Carr & Sheperd, 2011).
In 2002 the American Thoracic Society (ATS) published guidelines for the 6-minute walk test with the objective of standardizing the protocol to encourage its further application and to allow direct comparisons among different studies and populations. The American Thoracic Society guidelines include test indications and contraindications, safety measures, and a step-by-step protocol as well as assistance with clinical interpretation (Dunn et al., 2015).
Key components of the protocol include the test location, walkway length, measurements, and instructions. According to the American Thoracic Society protocol, the test should be performed on a flat, enclosed (indoor) walkway 30 m (just under 100 feet) in length. This protocol requires 180° turns at either end of the walkway and additional space for turning. The guidelines advise that shorter walkway lengths require more directional changes and can reduce the distances achieved. The influence of directional changes may be amplified in the stroke population, who characteristically have impaired balance, asymmetric gait patterns, and altered responses for turn preparation. Conversely, reducing the number of directional changes may increase the distance achieved (Dunn et al., 2015).
Body Weight-Supported Treadmill Training
Body weight-supported treadmill training (BWSTT) is an increasingly being used to encourage early walking following a stroke. It is a rehabilitation technique in which patients walk on a treadmill with their body weight partly supported. Body weight-supported treadmill training augments walking by enabling repetitive practice of gait (Takeuchi & Izumi, 2013).
In patients who have experienced a stroke, partial unloading of the lower extremities by the body weight-support system results in straighter trunk and knee alignment during the loading phase of walking. It can also improve swing1 asymmetry, stride length, and walking speed, and allows a patient to practice nearly normal gait patterns and avoid developing compensatory walking habits, such as hip hiking and circumduction2 (Takeuchi & Izumi, 2013).
1Swing phase of gait: during walking, the swing phase begins as the toe lifts of the ground, continues as the knee bends and the leg moves forward, and ends when the heel come in contact with the ground.
2Circumduction: a gait abnormality in which the leg is swung around and forward in a semi-circle. The hip is often hiked up to create enough room for the leg to swing forward.
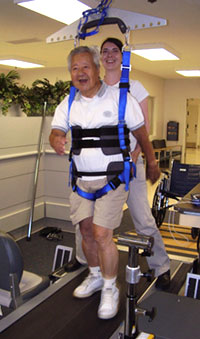
Example of a body-weight supported treadmill. Source: NIH, 2011.
Treadmill walking allows for independent and semi-supervised practice, for those with more ability, as well as improving aerobic capacity and increasing walking speed and endurance. The very early practice of assisted over-ground and harness-supported treadmill walking is probably critical to good post-discharge functional capacity in terms of both performance and energy levels (Carr & Shepherd, 2011).
The Locomotor Experience Applied Post Stroke (LEAPS) trial—the largest stroke rehabilitation study ever conducted in the United States—set out to compare the effectiveness of the body weight-supported treadmill training with walking practice. Participants started at two different stages—two months post stroke (early locomotor training) and six months post stroke (late locomotor training). The locomotor training was also compared to a home exercise program managed by a physical therapist, which was aimed at enhancing flexibility, range of motion, strength, and balance as a way to improve walking. The primary measure was improvement in walking at 1 year after the stroke (NINDS, 2011).
In the LEAPS trial, stroke patients who had physical therapy at home improved their ability to walk just as well as those who were treated in a training program that requires the use of a body weight-supported treadmill device followed by walking practice. The study, funded by the NIH, also found that patients continued to improve up to 1 year after stroke—defying conventional wisdom that recovery occurs early and tops out at 6 months. In fact, even patients who started rehabilitation as late as 6 months after stroke were able to improve their walking (NINDS, 2011).
“We were pleased to see that stroke patients who had a home physical therapy exercise program improved just as well as those who did the locomotor training,” said Pamela W. Duncan, principal investigator of LEAPS and professor at Duke University School of Medicine. “The home physical therapy program is more convenient and pragmatic. Usual care should incorporate more intensive exercise programs that are easily accessible to patients to improve walking, function, and quality of life.”
Robotic Gait Training Devices
Several lower-limb rehabilitation robots have been developed to restore mobility of the affected limbs. These systems can be grouped according to the rehabilitation principle they follow:
- Treadmill gait trainers
- Foot-plate-based gait trainers
- Over-ground gait trainers
- Stationary gait trainers
- Ankle rehabilitation systems
- Stationary systems
- Active foot orthoses (Díaz et al., 2011)
The Lokomat System
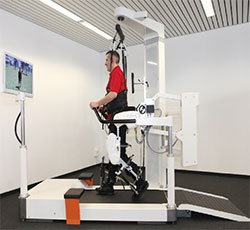
Source: Diaz et al., 2011.
Many robotic systems have been developed aiming to automate and improve body weight-assisted treadmill trainers as a means for reducing therapist labor. Usually these systems are based on exoskeleton type robots in combination with a treadmill. One such system—the Lokomat—consists of a robotic gait orthosis and an advanced body weight-support system, combined with a treadmill. It uses computer-controlled motors (drives) that are integrated in the gait orthosis at each hip and knee joint. The drives are precisely synchronized with the speed of the treadmill to ensure a precise match between the speed of the gait orthosis and the treadmill (Diaz et al., 2011).
The LokoHelp is another device developed for improving gait after brain injury. The LokoHelp is placed in the middle of the treadmill surface, parallel to the walking direction and fixed to the front of the treadmill with a simple clamp. It also provides a body weight-support system. Clinical trials have shown that the system improves the gait ability of the patient in the same way as the manual locomotor training; however, the LokoHelp required less therapeutic assistance and thus therapist discomfort is reduced. This fact is a general conclusion for almost all robotic systems to date (Díaz et al., 2011).
Over-ground gait trainers consist of robots that assist the patient in walking over ground. These trainers allow patients to move under their own control rather than moving them through predetermined movement patterns. The KineAssist is one robotic device used for gait and balance training. It consists of a custom-designed torso and pelvis harness attached to a mobile robotic base. The robot is controlled according to the forces detected from the subject by the load cells located in the pelvic harness (Díaz et al., 2011).
The ReWalk Robotic Suit
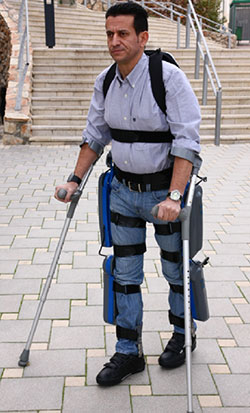
Source: Diaz et al., 2011.
ReWalk is a wearable, motorized quasi-robotic suit that can be used for therapeutic activities. ReWalk uses a light, wearable brace support suit that integrates motors at the joints, rechargeable batteries, an array of sensors, and a computer-based control system. Upper-body movements of the user are detected and used to initiate and maintain walking processes (Díaz et al., 2011).
The capacity of robots to deliver high-intensity and repeatable training make them potentially valuable tools to provide high-quality treatment at a lower cost and effort. These systems can also be used at home to allow patients to perform therapies independently, not replacing the therapist but supporting the therapy program. However, despite the attractiveness of robotic devices, clinical studies still show little evidence for the superior effectiveness of robotic therapy compared to current therapy practices, although robotics have been shown to reduce therapist effort, time, and costs (Díaz et al., 2011).