Pain plays an important in the survival of all animals. It acts as a signal, alerting us to potential tissue damage, and leads to a wide range of actions to prevent or limit further damage.
Physiologically, pain occurs when sensory nerve endings called nociceptors (also referred to as pain receptors) come into contact with a painful or noxious stimulus. The resulting nerve impulse travels from the sensory nerve ending to the spinal cord, where the impulse is rapidly shunted to the brain via nerve tracts in the spinal cord and brainstem. The brain processes the pain sensation and quickly responds with a motor response in an attempt to cease the action causing the pain.
Nociceptive Pathways
The classic nociceptive pathway involves three types of neurons:
- Primary sensory neurons in the peripheral nervous system, which conduct painful sensations from the periphery to the dorsal root of the spinal cord
- Secondary sensory neurons in the spinal cord or brainstem, which transmit the painful sensation to the thalamus
- Tertiary sensory neurons, which transmit the painful sensation from the thalamus to the somatosensory areas of the cerebral cortex.
Sensory Nerves Entering the Spinal Cord
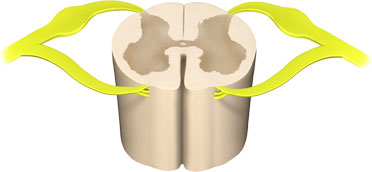
A section of a lumbar vertebra showing the sensory nerves (in yellow, with bulge) entering the dorsal part of the spinal cord. Illustration provided by 3DScience.com. Used by permission.
There are two major classes of nerve fibers associated with the transmission of pain:
- Unmyelinated C fibers
- Myelinated A-delta fibers
Destinations of the Spinothalamic and Spinoreticular Tracts in the Brain
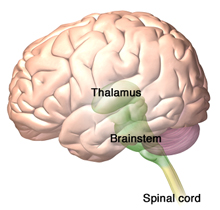
The thalamus is the destination of spinothalamic tract—the sensory pathway responsible for processing pain, temperature, and crude touch. The brainstem reticular formation, which forms a diffuse, central core within the brainstem is the destination of the spinoreticular tract. Source: 3DScience.com. Used by permission.
The C fibers are small and conduct impulses slowly. They respond to thermal, mechanical, and chemical stimuli and produce the sensation of dull, diffuse, aching, burning, and delayed pain. A-delta fibers, which are myelinated and thus conduct impulses rapidly, respond to mechanical (pressure) stimulus and produce the sensation of sharp, localized, fast pain.
One of the most important central pain pathways is the spinothalamic tract, which originates in the spinal cord and extends to the thalamus. This spinal tract transmits sensory information related to pain, temperature, and crude touch.
Another prominent pathway is the spinoreticular tract, which is involved in nociceptive processing. The spinoreticular tract is similar to the spinothalamic tract in that it is excited by similar sensory fibers. Rather than ascending to the thalamus however, spinoreticular neurons terminate within the brainstem.
Mechanisms of Pain
Pain can be caused by a mechanical, chemical or inflammatory, or thermal mechanism. Pain of mechanical origin can be caused by acute trauma, injury, or overuse. It may be constant, variable, or intermittent in nature and is affected by movement and position.
Pain of chemical or inflammatory origin is associated with arthritis and other inflammatory disorders. It is often constant but responds to positioning, therapy, rest, and gentle movement. Medications are usually a part of the management regimen for chemical or inflammatory pain.
Pain of thermal origin is the result of excessive heat or cold.
If an acute pain sensation is intense enough, it can cause system-wide responses: increased alertness; focused attention; the suppression of feeding, sleep, and reproduction; and increased vascular tone, respiration, and blood sugar levels. If pain persists or becomes chronic it can even change the circuitry in the central nervous system.
Using Neuroimaging to Understand Pain
Our understanding of how the brain changes in response to chronic pain or to pharmacologic or other therapeutic interventions has been significantly improved as a result of neuroimaging techniques. Until the advent of these techniques, the living brain was largely invisible to clinicians and researchers. The development of computed tomography (CT) and, soon thereafter, magnetic resonance imaging (MRI), allowed researchers to look into the living brain and gain some understanding of the parts of the brain affected by certain types of pain. The development of positron emission tomography (PET) has allowed researchers, for the first time, to investigate neuronal activity throughout the entire brain (Casey, 2015).
Functional magnetic resonance imaging (fMRI), positron emission tomography (PET), magnetoencephalography (MEG), and scalp electroencephalography (EEG) have been used to study the neural bases of pain. Other magnetic resonance-based measures such as diffusion tensor imaging, spectroscopy, and volumetric imaging are being used to assess pain-related changes in the brain’s wiring, chemistry, and structure; this will help gain further insights into the neurobiology of pain, particularly chronic pain (Lee & Tracey, 2013).
As a result, we now know that pain sensation is more complex than previously thought and involves diverse regions of the brain. Imaging techniques have allowed us to understand that pain results from activation of a number of brain regions such as the amygdala, insula, or the anterior cingulate cortex. We are learning that pain is a result of complex interactions between the immune, nervous (both CNS and autonomic nervous system), and endocrine systems (Zouikr et al., 2016).
In a study of patients with chronic low back pain, neuroimaging showed significant differences in brain function. Compared to healthy controls, chronic low back pain patients showed activation in pain-related brain regions during administration of experimental pain, differences in activation during emotional decision-making tasks, and changes in specific brain regions during a simple visual attention task (Kong et al., 2013).
Other imaging studies have reported structural changes in the brains of patients with chronic low back pain. One study found that these patients showed 5% to 11% less neocortical gray matter volume than control subjects and that the decreased volume was related to pain duration. These studies indicate that chronic low back pain is also associated with structural pathologic changes in the brain (Kong et al., 2013).
Neuroimaging studies are also being used to predict which patients might transition from acute to chronic pain. Using MRI scans in people with acute low back pain, researchers at Northwestern University Feinberg School of Medicine found abnormalities in the white matter in areas of the brain associated with the processing of pain and emotion. After one year, study participants were scanned again, and those with persistent pain showed the same abnormalities identified at the onset of the injury. In patients with ongoing low back pain, these abnormalities may be an indication of which patients will go on to develop chronic low back pain (Paul, 2013).
In patients with chronic pain, a number of research groups have reported significant changes in pain processing at a functional level including allodynia* (a stimulus not normally painful is perceived as painful), functional plasticity, and alterations in basic processes in the brain and brainstem. Alterations in neurotransmitters have also been reported in chronic pain patients using magnetic resonance spectroscopy. Such approaches have been applied to migraine, back pain, and spinal cord injury. The approach can be used to define neuronal and axonal markers, including specific metabolites such as glutamate, aspartate, glycine, and GABA (Borsook et al., 2007).
*Allodynia is a clinical feature of neuropathies, complex regional pain syndrome, post-herpetic neuralgia, migraine, and fibromyalgia.
At a macroscopic level, a number of studies have shown changes in volume in brain regions in patients with chronic neuropathic pain, complex regional pain syndrome, and fibromyalgia. This insight has been seminal in transforming our approach and thinking on chronic pain, since these changes indicate the potential of chronic pain being a neurodegenerative disease. At a microscopic level, changes in dendritic spine density or alterations in neuronal count have been observed in pain and stress. Such changes also have implications for the development of co-morbid disease such as depression (Borsook et al., 2007).
Sensitization
We can become sensitized to pain. Sensitization is a neurophysiologic term that describes the increased responsiveness of nociceptive neurons (the pain pathways become more sensitive). This can include a drop in the threshold for activating nociceptors and an increase in the frequency of firing for all stimuli (IASP, 2012). Hyperalgesia (exaggerated responses to stimuli) and allodynia (see above) are clinical markers used to detect the presence of sensitization (Zouikr et al., 2016).
In sensitization, stimuli that would not normally cause pain, such as the light touch of a sheet on the skin, can cause intense pain. Sensitization can also cause tenderness and soreness, particularly when deep tissue such as joints and hollow viscera are affected.
There are two types of sensitization: peripheral and central. Peripheral sensitization occurs in response to the release of inflammatory molecules such as histamine, prostaglandins, and pro-inflammatory cytokines. These substances sensitize (increase the excitability of) nociceptors by creating an “inflammatory soup” environment that enhances pain sensitivity by reducing the threshold of nociceptors activation (Zouikr et al., 2016). Under normal circumstances, peripheral hypersensitivity returns to normal when inflammation subsides or the source of the injury is removed (Kyranou & Puntillo, 2012).
In central sensitization, nociceptive-specific neurons may progressively increase their response to repeated non-painful stimuli, develop spontaneous activity, and increase the area of the body that is involved with the pain. The hyperalgesia of central sensitization usually develops as part of ongoing pathology (ie, damage to peripheral or central nerve fibers, cancer, rheumatoid arthritis) and is considered maladaptive (Kyranou & Puntillo, 2012).
Physiologic Effects of Unrelieved Pain
Unrelieved pain is a stressor that can lead to physiologic changes and negative effects on the endocrine, cardiovascular, gastrointestinal, and immune systems. The endocrine system reacts to unrelieved pain by releasing an excessive amount of hormones, ultimately resulting in carbohydrate, protein, and fat catabolism, poor glucose utilization, and other harmful effects. This reaction combined with inflammatory processes can produce weight loss, tachycardia, increased respiratory rate, fever, shock, and death.
The cardiovascular system responds to the stress of unrelieved pain by activating the sympathetic nervous system. Following a surgical procedure, for example, this can include hypercoagulation and increased heart rate, blood pressure, cardiac workload, and oxygen demand. Since the stress response causes an increase in sympathetic nervous system activity, intestinal secretions and smooth muscle sphincter tone increase, and gastric emptying and intestinal motility decrease. This response can cause temporary impairment of gastrointestinal function and increase the risk of ileus (intestinal obstruction). Aggressive pain control may be needed to reduce these effects and prevent thromboembolic complications.
Unrelieved pain may be especially harmful for patients with metastatic cancers. Stress and pain can suppress immune function, including the natural killer cells that play a role in preventing tumor growth and controlling metastasis.